Amazon today provided an update on Project Kuiper, its initiative to increase broadband access through a constellation of 3,236 low-Earth orbit (LEO) satellites. The team released information on the Ka-band phased array antenna for its low-cost customer terminal, signaling another milestone in the company’s efforts to provide fast and affordable broadband access to communities around the world.
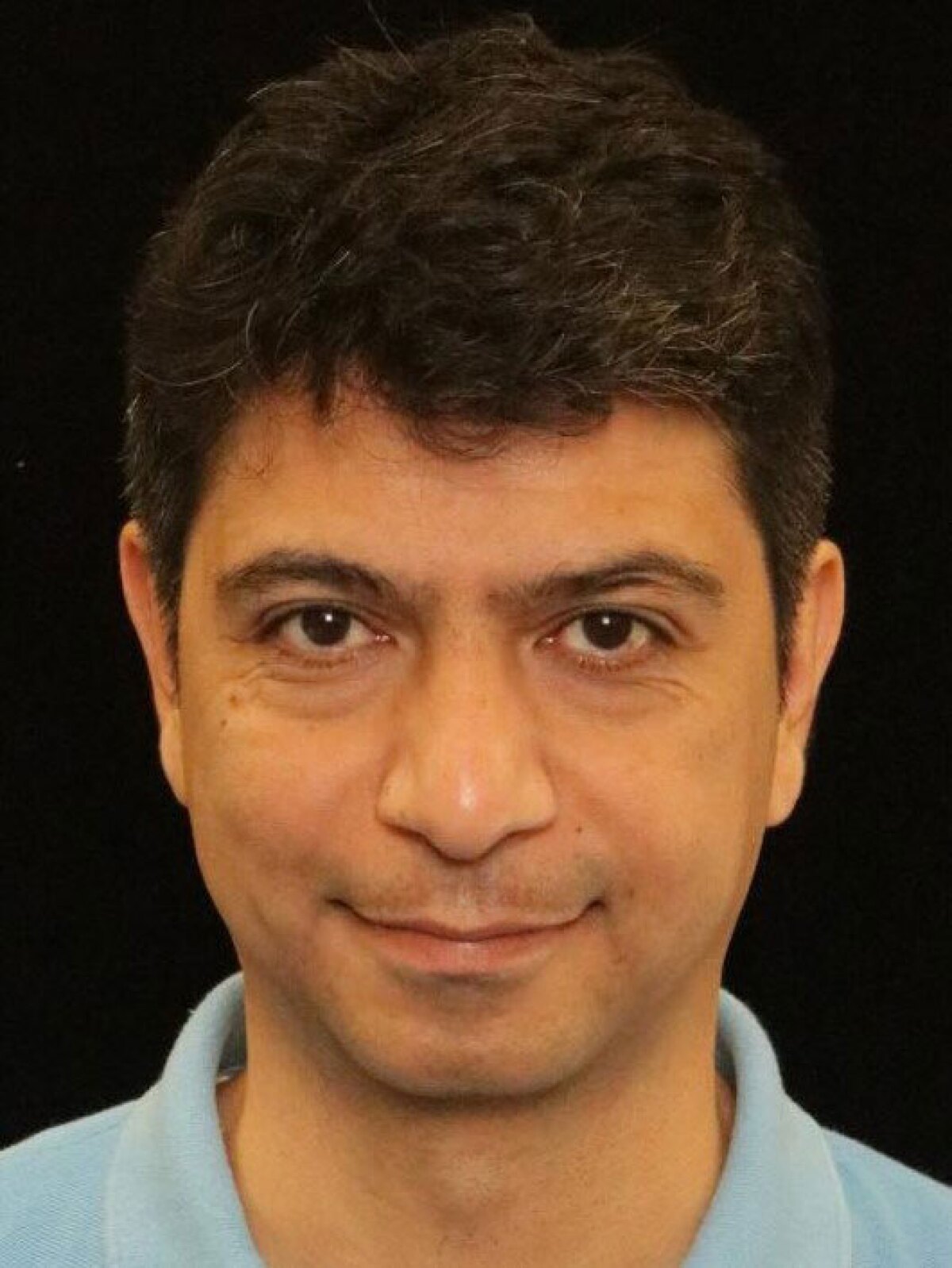
The prototype antenna is based on a new architecture designed and developed by the Project Kuiper team. The initial prototype is delivering speeds of up to 400 Mbps, despite a form factor that is approximately 12 inches in diameter and significantly lighter than legacy antenna designs. The reduction in size and complexity will allow Amazon to reduce production costs, contributing to the team’s goal of providing customers a terminal that is affordable and easy to install.
The antenna design and manufacturing effort is led in-house by the Project Kuiper team at Amazon. In advance of today’s announcement, Amazon Science asked Nima Mahanfar, senior manager of antenna development for Project Kuiper, about designing and developing the antenna, the science and engineering challenges his team encountered, tradeoffs the team had to wrestle with, and more.
The Kuiper prototype antenna is smaller and lighter than legacy Ka-band antennas. How did your team achieve that goal?
The key advancement was combining transmit and receive phased-array antennas into one aperture. This can be done in other frequency bands, but Project Kuiper plans to operate in Ka-Band, which has transmit and receive frequencies that are much further apart from one another. This makes it difficult, nearly impossible in fact, to combine transmit and receive into one aperture. Phased arrays are a class of radiating system, where multiple antennas — it could be two, it could be thousands— are on the same aperture, creating a focused beam of radio waves. The distance between the antennas — or the relation between these antennas — is decided by the frequency. If the frequencies are close to each other, as with Ku-Band, you can combine the transmit and receive function into one and it works. When the frequencies are far apart, as with Ka-Band, it’s much more difficult to utilize the same lattice for both. This has never been done before — until now.
Our design involves hundreds of antennas in each aperture, with receive antennas operating at 18 to 20 gigahertz (GHz) and transmit antennas operating at 28 to 30 GHz. Our breakthrough came from the realization that we could get to a single lattice by looking at each antenna element uniquely — helping reduce the size and cost of our entire terminal.
What were the science and engineering tradeoffs your team had to wrestle with in developing this new architecture, and what tenets guided your team’s work?
There were several, but I’ll focus on two key ones.
One is balancing the tradeoff between the transmit and receive functionality. From a customer obsession perspective, we realized that what affects the customer most is the receive function. In other words, we typically receive more information than we transmit. So we always err on the side of improving receive performance. On the transmit side, if you compromise performance, you can always increase the transmitted power by a little.
The second tradeoff relates to how easy our antenna is to manufacture. If our design was overly complex, it couldn’t be built affordably, or it couldn’t be scaled in production. We had to ask ourselves, at what point does this combined aperture become twice as complex as the single aperture, and would it still make sense? Our objective was to ensure our antenna was mass producible by mainstream circuit board manufacturers, allowing us to take advantage of economies of scale and produce millions at low cost. We had to keep our design as simple as possible to satisfy this objective, and this is an area where collaboration between scientists and manufacturing and hardware engineers was so important.
You received your PhD in high-frequency electronics and microwaves from the Université de Limoges and have been working in this field for a couple of decades. What are some of the key advancements that make Project Kuiper viable today? What are some of the interesting science and engineering challenges still left to address?
There are several important trends that make a project like this possible, and interestingly, few of them have to do with aerospace and satellite technology.
One is silicon. CMOS [complementary metal oxide semiconductor] technology has improved significantly, is available at a low cost, and can operate at higher and higher frequencies. Components that were once a luxury and confined primarily to the space and military industries, now are readily available and cost just a few dollars, or even cents. That has opened up this whole area for exploration.
The second trend comes from cellular technology and the cloud. As adoption and demand for these technologies has increased, we’ve seen higher frequency materials, and components available at larger scale and lower cost. At one time, building a printed circuit board at 30GHz was a niche thing. There were only a couple of manufacturers in the United States who could do this. They were expensive and they didn’t scale. Right now with 5G and even 4G cellular technology, basically we have more and more mass production of radio frequency (RF) at scale and at very high frequencies and customers are benefitting from this.
So those are the two technology trends that are helping us innovate easier, better and cheaper. The whole area of RF used to be exclusive to the space industry, and the military. That’s not the case anymore, and that’s great news for engineers like myself.
As for the challenges left to solve, I feel we can make phased array technology even more affordable. Not just by buying cheaper materials, but by developing new technologies and architectures that could be fundamentally different from today’s approach. We should be open-minded about the possibilities, and we are pursuing many of them already.
Another relates to the phased array technology we are building for our satellites in space, where the challenges are a little different. Cost is still important, but more importantly, we want to reduce watts per gigabits per second. Solving power challenges in space is hard, and dissipating the heat from that power is even harder. There’s no air to cool it. So having a low-power system that can provide many gigabytes of service to customers is key. How can we reduce the power consumption of these space-borne phased arrays? That’s one of the other big challenges facing anyone deploying phased array antennas in low earth orbit.
What type of scientist and engineer are you seeking to help address those challenges?
We’re seeking individuals with strong, fundamental science skills who understand the physical limits of what’s possible, and can deliver performance to that physical limit, or who can explain the imperfections for why we can’t. We want to deliver the best possible performance at the lowest possible price for our customers. To do this, we need to understand what possible based on physics rather than an arbitrary limit based on what’s been achieved in legacy systems.
Project Kuiper is made up of engineers and scientists with decades of experience in satellite communications, many of whom hold multiple patents for their work. Most of the folks I work with are PhDs, many in the field of electromagnetics, who aren’t fearful of doing original work. This is particularly germane for phased array technology where we’ve shifted to using printed circuit board technology versus expensive ceramics and other materials. So we’re seeking individuals who can recognize science projects from the real thing, and can then build advanced designs that come close to the physical limits of what’s possible. If this resonates with any of your readers, I hope they will visit our jobs page.